Thank you for visiting nature.com. You are using a browser version with limited support for CSS. To obtain the best experience, we recommend you use a more up to date browser (or turn off compatibility mode in Internet Explorer). In the meantime, to ensure continued support, we are displaying the site without styles and JavaScript.
Carousel with three slides shown at a time. Use the Previous and Next buttons to navigate three slides at a time, or the slide dot buttons at the end to jump three slides at a time. Sodium Dimethyldithiocarbamate
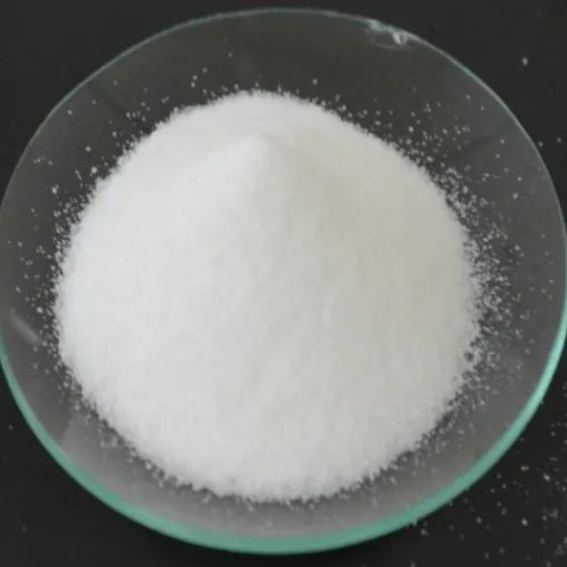
Joo Sung Kim, Jung-Min Heo, … Tae-Woo Lee
Benbing Shi, Xiao Pang, … Zhongyi Jiang
Ming Xu, Xuetao Qin, … Ding Ma
Dongmin Wang, Cai Zhai, … Gengwen Tan
Chao-Yang Wang, Teng Liu, … Brian D. McCarthy
Gavin Harper, Roberto Sommerville, … Paul Anderson
Changshun Chen, Jianxin Chen, … Wei Huang
Liguang Wang, Tongchao Liu, … Jun Lu
Xu Li, Xavier Isidro Pereira-Hernández, … Jingyue Liu
Scientific Reports volume 12, Article number: 15634 (2022 ) Cite this article
This paper threw some light on the behavior of Sodium N,N-Dimethyldithiocarbamate as an electrolyte. The effect of solvents on the conductance of this salt would be discussed via measurements of Λo, ao and KA, since it can be assumed that the different solvents have a little chance to impose great variations on the solvation processes. The conductance method was chosen as a tool to illustrate the electrolyte-solvent interactions. Fuoss–Onsager equation would be tested using Sodium Dimethyldithiocarbamate in presence of dimethylformamide solvent at different temperatures. The conductance of dilute solutions of Sodium N,N-Dimethyldithiocarbamate is measured in Dimethylformamide, at different temperatures (25, 30, 35 and 40 °C). Accurate values of Λo were obtained by applying the (Fuoss–Kraus–Shedlovsky) equation. Finally, the (Fuoss–Onsager) equation was solved to give the correct values of the constants Λo, J, KA and a° (the closest distance of approach) for Sodium N,N-Dimethyldithiocarbamate salt in Dimethylformamide solvent. Λo and a° (solvation) increase with increasing temperatures. Thermodynamic parameters (∆G°, ∆H°, ∆S° and ∆Es) of Sodium N,N-Dimethyldithiocarbamate in Dimethylformamide were calculated from conductance measurements, the activation energy (∆Es), the enthalpy change (heat of association) (∆H°) and the entropy change (∆S°) are positive, however The free energy change (∆G°) values was negative for Sodium N,N-Dimethyl dithiocarbamate in DMF systems studied with increasing the temperature.
The density is one of the thermodynamic properties of electrolyte solutions and the viscosity is the transport properties of electrolyte solutions. Both dispensable basic data to design of engineering and process optimization.
Sodium N,N-dimethylthithiocarbamate's are important organic compound used in many applications and uses as a disinfectant, corrosion inhibitor, coagulant, vulcanizing agent, chelating agent, and fungicide which may result in its release to the environment1. Sodium N,N-dimethylthithiocarbamate's used in water treatment, rubber industry and is a registered biocide for cutting oils and aqueous systems in industries such as leather tanning and paper manufacturing2. Also it used as an antimicrobial agent in paints3.
In general, thermodynamic and transport properties of electrolyte solutions have a great importance due to their wide applications in different chemical industries (e.g. electrochemical process like corrosion or electrolysis, environmental applications, hydrometallurgical process, separation techniques like seawater desalination, crystallization and extractive distillation, and production of energy sources. Measuring the transport properties as conductance at low concentrations helps in exploring the ionic solvation also helps in obtaining reliable values of conductance at infinite dilution, these properties are affected by size of ions, any modulation in the structure of the solvent and the strong ion–solvent interactions1,4,5.
The aim of this paper is to explain the behavior of Sodium N,N-Dimethyldithiocarbamate as an electrolyte. The effect of solvents on the conductance of this salt would be discussed via measurements of Λo, a° and KA, since it can be assumed that the different solvents have a little chance to impose great variations on the solvation processes. The conductance method was chosen as a tool to illustrate the electrolyte–solvent interactions. Fuoss–Onsager equation would be tested using Sodium Dimethyldithiocarbamate in presence of Dimethylformamide solvent at different temperatures (25, 30, 35 and 40 °C).
In addition, The variation of KA in Dimethylformamide solvent was explained, the electrostatic radius of the ions was calculated using the Stokes' equation and a comparison between the sum (R+ + R−) and the closest distance for approach between cation and anion (a°) would be discussed and the activation energy ∆Es and Thermodynamic parameters (∆G°, ∆H°, and ∆S°) were calculated in Dimethylformamide solvent at different temperatures (25, 30, 35 and 40 °C).
Salt was highly purified grade and used without extra purification. Where Sodium N,N-Dimethyl dithiocarbamate (NaS2CN (CH3)2) is Analar analytical reagent "BDH" as shown in Fig. 1.
The structure of Sodium N,N-Dimethyl dithiocarbamate10.
All glassware used were left over night in chromic acid, then washed with tap water, distilled water, conductivity water and finally steamed for about half an hour, and dried in an electric oven for 24 h.
Analar analytical reagent Dimethylformamide (BDH), was used without further purification. The specific conductance ° for Dimethylformamide was found to be (1.6–2.6 × 10–6) ohm−1 cm−1.
All solutions were prepared by weight. Salt is weighted by difference on a microbalance which reads to ± 0.1 mg. Dilution was carried out successively into the cell by siphoning the solvent by means of a weighing pipette. The salt was dissolved by carefully shaking the conductivity cell which is suspended in the water ultra-thermostat. The concentration of the solution was obtained by using the equation:
The cell constant was 0.1 cm−1 for dilute solutions. The measurement error of the conductivity meter used (± 1 digit) ≤ 0.5% and the reproducibility (± 1 digit) ± 0.1%.
The resistance of the solution was measured several time intervals (15 min) after throughly shaking.
The specific conductance of solute \(\vartheta\) could be calculated from the relation:
where r is the resistance of solution, \(\vartheta\) is the specific conductance of solute and could be calculated from the relation.
Different concentrations (C equiv. L−1) were prepared inside the cell using the weighing pipette as described before and the corresponding equivalent conductance values Λ, were calculated from the following equation:
where Λ is the equivalent conductance (ohm−1 equiv.−1 cm2), \(\vartheta\) is the specific conductance of solution (ohm−1 cm−1), C is the concentration (equiv. L−1).
Precise conductance measurements were repeated for Sodium N,N-Dimethyldithiocarbamate in Dimethylformamide (DMF) at different temperatures (25, 30, 35 and 40 °C). The chemical formula for this salt is [(NaS2CN (CH3)2)].The abbreviations of this salt is (Na.DMDTC).
The solvent parameters of Dimethylformamide (DMF) at 25, 30, 35 and 40 °C, used in the calculations, are shown in Table 16,7,8,9 where d is the absolute density at 25, 30, 35 and 40 °C, η is the viscosity at 25, 30, 35 and 40 °C, D is the dielectric constant at 25, 30, 35 and 40 °C.
Table 2 shows the \(\vartheta\) is the specific conductance of the pure solvents at different temperatures. The values of equivalent conductance Λ (ohm−1 equiv−1 cm2) corresponding to several values of concentration C in (equiv. per liter) were obtained for the salt.
A preliminary value of Λo (the equivalent conductance at infinite dilution) was estimated from Λ versus C1/2 plot as illustrated in Fig. 2. Accurate value of Λo was obtained from the (F.K.S) equation. Since a plot of 1/ΛS(z) versus S(z) f2CΛ as illustrated in Fig. 3 for DMF at 25, 30, 35 and 40 °C respectively should yield a straight line, the intercept then equals 1/Λo and the slope is 1/KD Λo2.
Conductance of Sodium N,N Dimethyl dithiocarbamate in Dimethylformamide at (a) 25, (b) 30, (c) 35 and (d) 40 °C.
(F.K.S) plots for Sodium N,N-Dimethyl dithiocarbamate in Dimethylformamide at (a) 25 °C, (b) 30 °C, (c) 35 °C and (d) 40 °C.
The three-parameter shown in the Eq. (4):
This theory was confirmed by the approximate agreement of ion sizes computed from Λo, J(a°) and KA.
The calculated values of the parameters Λo, J(a°) and KA were calculated using a computer program. The accuracies which is represented by the standard deviation values are ± 0.02 for Λo, ± 2 for J < 200, ± 5 for J (200–1000) and ± 10 for J > 1000.
Figure 4 show the variation of a° with J(a°), from which a° can be determined by interpolation. The results were shown in Table 4. The last column in this table illustrates the standard deviation σΛ which was calculated using Eq. (5):
where N is the number of experimental points.
Variation of J and a° of Sodium N,N-Dimethyl dithiocarbamate in Dimethylformamide at 25, 30, 35 and 40 °C.
It can be seen from Table 3 and Fig. 4 that Λo values increases for Sodium N,N-Dimethyldithiocarbamate from DMF at different temperatures. The values of ao (solvation) also increases in case of using DMF at different temperatures. This means that the ionic equivalent conductance becomes the main factor controlling the extent of ion-pairing.
In the present work for dilute solutions of Sodium N,N-Dimethyldithiocarbamate measured in DMF at (25, 30, 35 and 40 °C), the trend was that KA increases in the presence of DMF. It can be seen also from Table 4 that Λo, KA and ao increases, for Sodium N,N-Dimethyldithiocarbamate in DMF with increasing the temperatures, from 25 to 40 °C, according to ion–dipole interactions between solvents and ions.
The trend of KA in the present work was explained in the light of the U term as represented in Eq. (6) 11:
(ΔS/k) is the Entropy Boltzmann constant ratio which illustrates the probability of the orientation of the solvent molecules around the free ions and (Es/kT) is an energy relationship which includes the energy of the solvent molecules with respect to the free ions (i.e. ion–dipole interaction) and ion-pairs.
It can be seen from Table 4 that U term increases with increasing the temperatures for Sodium N,N-Dimethyldithiocarbamate in DMF from 25 to 40 °C, i.e. the entropy is more predominant than the term of ion–dipole. Finally, solvent separated ion-pair model has been applied12. In this model a multiple step association is suggested, i.e. solvent separated and contact ion-pair can be illustrated in the following Fig. 5:
Where y is the number of escaping solvent molecules from solvation Thus, the association constant KA can be given by the expression:
where KA is obtained from the conductance measurements and since
Then K2 can be calculated from Eq. (10). The results compiled in Table 4 indicated that in case of Sodium N,N-Dimethyl-dithiocarbamate in DMF at different temperatures, K1 increases with increasing the temperatures, i.e. ion-pair preferred the solvated form (case I) than the de-solvated form (case II).
The electrostatic radius (R+ + R−) was given by Stokes' equation:
where ηo is the viscosity of pure solvent and λo− is obtained from the intercept of the straight line, resulting from the plots of Walden product Λo ηo versus the reciprocal of the molecular weight as previously discussed13, while λo+ for sodium was represented by the value of Λo of the Sodium N,N-Dimethyl dithiocarbamate salt.
From the data in Table 5, it could be seen that the values of a° were greater than electrostatic radii (R+ + R−) obtained from Stokes equation in case of DMF at different temperatures from 25 to 40 °C. This was due to the solvation of ions.
Thermodynamic parameters (∆H°, ∆G°, ∆S°) and activation energy (∆Es) were calculated to explain the limiting equivalent conductance (Λ0) and ion association constant (KA) of Sodium N,N-Dimethyl dithiocarbamate in DMF at different temperatures using conductance measurements. Three parameters were evaluated by using Fuoss-Onsager equation. The solvent parameters of DMF at different temperatures (25, 30, 35 and 40 °C) were given in Table 1.
It is evident from Table 6 that, the values of Λ0 increase regularly with increasing the temperature for Sodium N,N-Dimethyl dithiocarbamate salt, indicating less solvation or higher ions mobility in the solvent system studied. This is due to the increase in the results of thermal energy in greater bond breaking and the variation in rotational, vibrational and translational energy of the molecules, which leads to higher frequency and higher ions mobility. In addition, it is clear that the association constant (KA) values increase with increasing the temperature, due to the decrease in dielectric constant of the medium14.
Since the measurements of the conductance of an ion depend on its mobility, it is reasonable to treat the data of the conductance which similar to the one that employed for the processes taking place with change of temperature (9), i.e.
where the symbol A is the frequency factor, R is the constant of the ideal gas and ∆ES is the activation energy of Arrhenius of transport processes.
The values of ∆ES computed from the slope (− ∆ES/2.303R) of the plot of log Λ0 versus 1/T and the values recorded in Table 6 and Fig. 5. From the Table 6, the activation energy (∆Es) is positive for Sodium N,N-Dimethyl dithiocarbamate in DMF systems studied. The values increase in case of DMF which indicates the higher mobility of the ions in the solution and hence higher Λ0 values. The free energy change ∆G° for the association process is calculated from Eq. (13) 15
it is evident from Table 6 and Figs. 6 and 7 that, the free energy change (∆Go) values are negative for Sodium N,N-Dimethyl dithiocarbamate in DMF was studied and this values agree with the values obtained from S.Pura16.
The multiple steps of association14.
The variation of log Λ0 versus 1/T for Sodium N,N-Dimethyl dithiocarbamate in Dimethylformamide at different temperatures.
The variation of log KA versus 1/T for Sodium N,N-Dimethyldithiocarbamate in Dimethylformamide at different temperatures.
This means that the association process is favored over the dissociation process in DMF system. The values of (∆Go) become more negative with increasing the temperature. The increase in (∆G°) values for Sodium N,N-Dimethyl dithiocarbamate salts favors the transfer of the released solvent molecules into bulk solvent and leads to a larger (∆G°) values. The strengthening to the interionic association at higher temperature is largely caused by a decrease in the permittivity of the solvent17.
The enthalpy change which is the (heat of association) (∆H°) obtained from the slope of the plot of log KA versus 1/T as shown in Fig. 7. The values of (∆H°) were calculated, where the slope equals (−∆H°/2.303R). The values of (∆H°) are positive which agree with Dash et.al.14.
The positive values of (∆H°) for Sodium N,N-Dimethyl dithiocarbamate salt shows that the association processes are endothermic in nature. Positive values and high values of (∆H°) attributed to the interaction between ions17.
The entropy change (∆S°) was calculated, from Gibbs–Helmholtz Eq. (14):
The values of (∆S°) are positive which agree with Dash et al.13 the positive values of (∆S°) for Sodium N,N-Dimethyl dithiocarbamate salt indicate the randomness of ions in all solvent systems studied. As presented in Table 6, values of (∆S°) were positive because of the decrease in the solvation of ion-pair compared to that of the free ion which agree with Bag et al.18. This may be attributed to increase in the degree of freedom upon association, mainly due to the release of solvent molecules this values agree with the values obtained19,20,21,22,25,26,27,28,29,30,31,32,33.
The main factors, which govern the standard entropy of ion association of electrolytes, are.
The ions size and shape.
The solvent molecules electrostriction around the ions and
The solvent penetration of the molecules inside the space of ions23,24.
The following conclusions arise from the work described here in:
The activation energy (∆Es) is positive for Sodium N,N-Dimethyl dithiocarbamate in DMF systems and is high due to the higher mobility of the ions in the solution and hence higher Λ0 values
The free energy change (∆G°) values is negative and this means that the association process is favored over the dissociation process in DMF system.
The values of (∆G°) are more negative with increasing the temperature.
The enthalpy change (heat of association) (∆H°) is positive and this means that the association processes are endothermic in nature and attributed to the interaction between ions.
The positive values of (∆S°) indicates the randomness of ions in all solvent systems studied due to the decrease of degree of solvation of ion-pair compared to that of the free ion. This may be attributed to the increasing in degree of freedom upon association.
The datasets used and analyzed during the current study are available from the corresponding author on reasonable request.
The number of escaping solvent molecules from solvation
The Entropy Boltzmann constant ratio which illustrates the probability of the orientation of the solvent molecules around the free ions
Equivalent conductance (ohm−1 equiv−1 cm2)
Specific conductance of solution (ohm−1 cm−1)
The absolute density (g L−1)
The specific conductance of the pure solvents at different temperatures
The equivalent conductance at infinite dilution
The viscosity of pure solvent
The viscosity of pure solvent
Arrhenius activation energy of transport processes
The enthalpy change (heat of association)
Verschueren, K. Handbook of Environmental Data on Organic Chemicals, vol. 1–2, 4th ed. 912 (Wiley, 2001).
US EPA. High Production Volume (HPV) Challenge Program. The HPV Voluntary Challenge Chemical List. Robust Summaries and Test Plans. (USEPA, Off Prevent Pest Tox Subst, Pollut Prevent Toxics, 2004).
McEntee, T. Kirk-Othmer Encycl Chem Technol, "chemie ingenieur Technik", vol. 14, 4th ed. 174–199 (Wiley, 1995).
Masood, S., Saeed, R. & Khan, S. R. Conduct metric and thermodynamic study of copper and nickel sulfate in aqueous methanol systems. J. Mater. Phys. Chem. 1, 69 (2013).
Anderko, A., Wang, P. & Rafal, M. Electrolyte solutions: from thermodynamic and transport property models to the simulation of industrial processes. Fluid Phase Equilib. 194, 123–142 (2002).
Safonova, L. P., Sakharov, D. V., Shmukler, L. E. & Kolker, A. M. Conductance studies of 1–1 electrolytes in N,N-dimethylformamide at various temperatures. Phys. Chem. Chem. Phys. 3, 819 (2001).
Pura, S. The effect of temperature on the equivalent conductivities and ion-association constants of some tris-(ethylenediamine)chromium(III) complexes in N,N-dimethylformamide and N,N-dimethylacetamide. J. Solut. Chem. 37, 351 (2008).
Chipperfield, J. R. Non-Aqueous Solvents. (Oxford University Press, 1999).
Marcus, Y. The Properties of Solvents. (Wiley, 1998).
https://pubchem.ncbi.nlm.nih.gov/compound/Sodium-dimethyldithiocarbamate.
Accascina, F., D’Aprano, A. & Triolo, R. Ion pairs and solvent-solute interaction. I. Conductance of lithium chlorate in water-dioxane mixtures at 25 degree. J. Phys. Chem. 71, 3469 (1967).
Evans, D. F. & Gardam, P. Hydration structure and diffusion of ions in supercooled water: Ion size effects. J. Phys. Chem. 73, 158 (1969).
EI-Hammamy , NH , Amira , MF , Abou EI-Enein , SA , Abd EI-Halim , FM ,.electrolytic behavior of acetylcholine halides & perchlorate in aqueous solutions.Indian J. Chem.Rev. 23A, 43 (1984).
Dash , UN , Mahatra , JR & Lal , BJ Mol.Liq.124 , 13 ( 2006 ).
Coetzee, J. F. & Ritchie, C. D. Solute-Solvent Interaction Vol. 2 (Marcel-Dekker, 1976).
Pura, S. Electrical conductivity of s-acetylthiocholine halides and perchlorate in ethanol at 30°C. J. Mol. Liq. 136, 64 (2007).
Kalugin, O. N., Agieienko, V. N., Otroshko, N. A. & Moroz, V. V. Russian “Ionic association and solvation in solutions of magnesium and nickel perchlorates in acetonitrile”. J. Phys. Chem. A 83(2), 231 (2009).
Bag, G. C., Singh, N. M. & Singh, N. R. J. Indian Chem. 68(6), 507–510 (2001).
El-Hammamy, N. H., El-Kholy, M. M., Kawana, A. I. & Ibrahim, G. A. Electrical conductance and ion pair formation of sodium diethyldithiocarbamate in acetonitrile-water mixtures at 25°C. J. Chem. Pharm. Res. 2(4), 1112 (2010).
El-Hammamy, N. H., El-Kholy, M. M., Ibrahim, G. A. & Kawana, A. I. Electrochemical studies on ion pairs formation of trimethylsulfonium halides in 2-propanol at different temperatures. J. Chem. Pharm. Res. 2(4), 984 (2010).
El-Hammamy, N. H., Kawana, A. I., El-Kholy, M. M., Amira, M. F. & Ibrahim, G. A. Electrochemical studies on ion pairs formation of sodium diethyldithiocarbamate in water. J. Alex. Pharm. Sci. 23, 79 (2009).
El-Hammamy, NH & El-Araby, HA Sesame husk as adsorbent for copper(II) ion removal from aqueous solution.Int.J. Innov.Sci.Res.29(2), 184–192 (2017).
Pura, S. & Atun, G. Conductometric study of ion association of hexaamminecobalt (III) complexes in ethanol+ water. J. Chem. Eng. Data 47, 1103 (2002).
Victor, P. J., Muhuri, P. K., Bas, B. & Hazra, D. K. Electrical conductivity and thermodynamic studies on trimethylsulfonium halides in methanol at different temperatures. J. Phys. Chem. B 103, 11227 (1999).
Darweesh, M. A. et al. A unique, inexpensive, and abundantly available adsorbent: Composite of synthesized silver nanoparticles (AgNPs) and banana leaves powder (BLP). Heliyon 8, e09279 (2022).
Darweesh, M. A. et al. Adsorption isotherm, kinetic, and optimization studies for copper (II) removal from aqueous solutions by banana leaves and derived activated carbon. S. Afr. J. Chem. Eng. 40, 10–20. https://doi.org/10.1016/j.sajce.2022.01.002 (2022).
Ahmed, A. M., Ayed, M. I., Aledkawy, M. A., Darweesh, M. A. & Elmelegy, E. M. Removal of iron, zinc, and nickel-ions using nano bentonite and its applications on power station wastewater. Heliyon 7, e063015 (2021).
Ahmed, A. M., Darweesh, M., Mahgoub, F. M. & Hammad, W. A. Adsorption kinetic study for removal of heavy metal from aqueous solution by natural adsorbent. DJ Eng. Chem. Fuel 4(1), 12–26. https://doi.org/10.18831/djchem.org/2019011002 (2019).
Ahmed, A. M., Aggor, Y. A., Darweesh, M. A. & Noureldeen, M. I. Removal of ferrous ions from city water by activated carbon prepared from palm fronds. DJ J. Eng. Chem. Fuel. 3(1), 46–63. https://doi.org/10.18831/djchem.org/2018011005 (2018).
Darweesh, M. A. & Salem, D. M. S. A. Removal of Cr+6 ions from wastewater in presence of quaternary ammonium salts. Int. J. Basic Appl. Chem. Sci. 2(1), 46–49 (2012).
Darweesh, M. A. Reduction of Cr6+ ions in presence of non toxic acid. Life Sci. J. 9(2) (2012).
Akhter, Y. & Darwish, M. Ultrasonic and interaction studies of amino acids in aqueous electrolytes Cu (II) nitrate and Ni (II) chloride solutions at 308 K. Invertis J. Sci. Technol. 5(3), 171–173 (2012).
Abdel-Rahman, H. H. & Darweesh, M. A. Removal of Cr+6 ions from wastewater in presence of some nontoxic amino acids. Egypt. J. Chem. 49, 19–34 (2006).
The author thanks the project Laboratory, Faculty of science, Alexandria University, Egypt and faculty of engineering Tanta University, Egypt for their assistance during this work. And also, we would like to thank the Egyptian Knowledge Bank (EKB) for funding this article.
Open access funding provided by The Science, Technology & Innovation Funding Authority (STDF) in cooperation with The Egyptian Knowledge Bank (EKB). The funding was provided by Science and Technology Development Fund (Springer Nature OA agreements for Egypt).
Faculty of Engineering, Tanta University, Tanta, Egypt
W. A. Hammad & M. A. Darweesh
Faculty of Science, Alexandria University, Alexandria, Egypt
N. H. El-Hammamy & M. H. Morshidy
Tabuk University, Tabuk, Saudi Arabia
You can also search for this author in PubMed Google Scholar
You can also search for this author in PubMed Google Scholar
You can also search for this author in PubMed Google Scholar
You can also search for this author in PubMed Google Scholar
You can also search for this author in PubMed Google Scholar
Professor N.H.E.-H. God bless his soul. W.A.H. work the experimental work. M.A.D. wrote the idea and revision the final version of paper. Kh.M.A. wrote the first version of paper. N.H.E.-H. revised some experimental data before his death. M.H.M. wrote the final version of paper.
Correspondence to W. A. Hammad.
The authors declare no competing interests.
Springer Nature remains neutral with regard to jurisdictional claims in published maps and institutional affiliations.
Open Access This article is licensed under a Creative Commons Attribution 4.0 International License, which permits use, sharing, adaptation, distribution and reproduction in any medium or format, as long as you give appropriate credit to the original author(s) and the source, provide a link to the Creative Commons licence, and indicate if changes were made. The images or other third party material in this article are included in the article's Creative Commons licence, unless indicated otherwise in a credit line to the material. If material is not included in the article's Creative Commons licence and your intended use is not permitted by statutory regulation or exceeds the permitted use, you will need to obtain permission directly from the copyright holder. To view a copy of this licence, visit http://creativecommons.org/licenses/by/4.0/.
Hammad, W.A., El-Hammamy, N.H., Morshidy, M.H. et al. Electrical conductivity and thermodynamic studies on Sodium Dimethyldithiocarbamate in non aqueous solvents Dimethylformamide (DMF), at different temperatures. Sci Rep 12, 15634 (2022). https://doi.org/10.1038/s41598-022-18849-7
DOI: https://doi.org/10.1038/s41598-022-18849-7
Anyone you share the following link with will be able to read this content:
Sorry, a shareable link is not currently available for this article.
Provided by the Springer Nature SharedIt content-sharing initiative
By submitting a comment you agree to abide by our Terms and Community Guidelines. If you find something abusive or that does not comply with our terms or guidelines please flag it as inappropriate.
Scientific Reports (Sci Rep) ISSN 2045-2322 (online)

Perfluoropolyether Pfpe Sign up for the Nature Briefing newsletter — what matters in science, free to your inbox daily.